Digital Energy Estimation Tool
About the project
In this research project the research team has developed a unique parametric design-based methodology for estimating total energy use in a building utilising BIM (Building Information Modelling) frameworks and protocols. Results from the work have indicated that embodied energy can be much more significant in the first few phases of the buildings life cycle, and material selection can be addressed within a parametric model.
In addition, the project had a unique multidisciplinary research approach to show how technologies such as BIM and virtual reality can be used to communicate the message of enhancing the performance of the built environment and the cities and communities it serves. The project achieved the development of data rich 3D construction templates that addressed more efficiently the decision making potential of BIM processes. A final outcome of the work was the realization of how such aspects such as energy and carbon in the built environment assets can be visualised with virtual reality to give it greater acceptance.
The Challenge
According to the Energy Performance of Buildings Regulation [1], in new buildings, energy analysis should be carried out at an early stage of the design process where more opportunities are available to optimise the energy consumption. Therefore, estimating energy consumption in the early stages of building design has received a substantial importance today for energy and emission reduction efforts. Even though, many developments such as energy modelling tools, models, software and frameworks have been developed to simplify the life cycle energy assessment of buildings, only fewer researchers have attempted to estimate both operational and embodied energy of buildings.
The first challenge identified is the complexity and time-consuming nature of mapping the Life Cycle Inventory [LCI] input data with building material quantities. Estimating whole life cycle energy consumption during early design stage of a building is a complicated task which depends on number of factors such as building characteristics, materials use, energy systems characteristics, control and maintenance, weather parameters, and behaviour of occupants. Therefore, achieving an accurate estimation of total energy consumption is challenging. The second challenge is the lack of interoperability between BIM model and LCA tools which limited the role of BIM model in the framework as just an automatic material take-off. The third challenge is determining an adequate granularity and development process for building BIM model.
This project proposes a BIM enabled approach to predict total energy consumption of commercial and residential buildings in the UK. It enables the generation of real-time energy data, both embodied energy from building components through 3-D computing paradigm at an early building design stage, coupled with operational energy input, bringing a new approach to total life analysis of energy and carbon. Such data will allow more accurate prediction of impacts and material substitution by linking both operational and embodied energy in one tool.
The Solution
First, a systematic literature review was carried out to identify the challenges and limitations of BIM and energy estimation. Consequently, as illustrated in Figure 1, the identified challenges and limitations were taken into consideration and used as the basis for developing a solution. The proposed framework should consider a user-friendly interface, minimise the several manual entries, and not require high experienced LCA practitioner to deal with assigning the right sustainability data for materials and systems used.
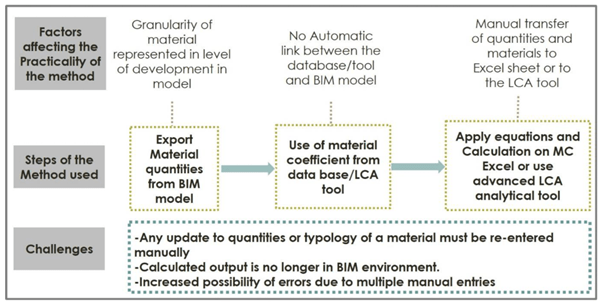
Figure 1
Measurement of the overall energy impact of all components is almost impossible as a building might have 1000 plus items. Therefore, a scope had to be set to identify the parameters of the components that can be measured. For embodied energy, this project focused on material parts of the building which were termed as “energy hot spots” due to their larger energy consumption, including substructure, superstructure and internal finishes. External works were disregarded due to unavailability of information. Operational energy is measured either using standard coefficients for consumption or using established measurement such dynamic modelling tools. Building type, whether domestic or commercial, determined the type of methods used to measure operational energy, which helped give precise reflections of energy consumption.
The research team then developed a bespoke parametric design tool which addresses both operational and embodied energy based on established benchmarks already in use in the industry such as, BREDEM CIBSE. The ultimate aim was to design a tool prototype that could be changed and modified by professionals operating within the AEC sectors.
In order to get an overall account of the role of built assets within the built environment, three different building types were chosen, two domestic and one commercial building, in order to get different representations of construction materials and operating patterns.
To create a customised tool, Grasshopper was used. Grasshopper is a platform that is widely used by designers today. It is a free open source where users can customise the tool based on their needs and contribute to the source code. Also, Honeybee was used to provide energy and daylight modelling. Honeybee is the extension of Ladybug which allows users to work with validated energy and daylighting engines such as EnergyPlus, Radiance and Daysim. Integration with the parametric tools of Grasshopper allows for almost instantaneous feedback on design modifications, and as it runs within the design environment, the information and analysis are interactive. The analysis is done in four steps in Honeybee, which are; preparing simulation geometry, checking the input file, running the simulation(s) and visualising the results.
Figure 2 shows the process of linking building geometry to operational costs and embodied carbon. First, a 2D single line plan drawing was modelled in Rhino and then linked to Grasshopper. The three-dimensional model was modelled in grasshopper with variable range of WWR and space heights. All building elements, ground, walls, roof, windows, etc. were separately analysed in terms of areas. The material decision and geometrical variables; WWR and space height; have instantaneous feedback from the embodied energy and operational cost, hence the total cost estimation of the building over a lifetime span for each design and material proposal. The material definition depends on a selection from the honeybee and therm libraries. Each surface is composed of single or multiple layers of materials to form a component. The selected material is changed from a drop-down menu using an item selector component (material decisions). These material decisions were scripted to affect both embodied and operational energy analysis.
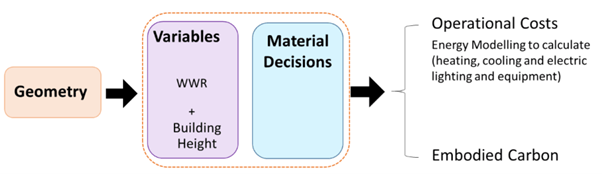
Figure 2
For LCA analysis, an open-source plugin into Grasshopper for LCA called Bombyx was used. Bombyx plugin allows for simplified whole building Life Cycle Assessment (LCA). Connecting the geometry
drawn in rhino with named layers with corresponding geometry containers in grasshopper to analyse the area and then specify thickness, material and reference service life for each layer. The impact of layers, elements, components and the whole building is calculated per year and then the Global Warming Potential [GWP].
Figure 3 illustrates the overall roadmap of the parametric model as developed on Grasshopper.
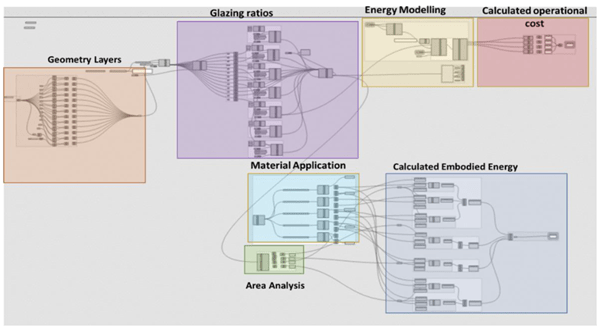
Figure 3
Key findings:
- Widening understanding of embodied energy and its impact on the built environment within a building can vary in their contribution to embodied energy, and certain material can be classified as energy hotspots.
- A framework has to be developed for measuring embodied energy impact as not all components can be measured.
Embodied energy contribution can play an important role in the first few years of the building lifecycle. - Established and adopted a framework to identify the parameters that can actually be measured.
Within a single parametric model, whole energy – both operational and embodied – can potentially be analysed in a VR environment.
External Partners: Centre for Digital Built Britain
Research Dates: 2019 – 2022
Lead Academic on This Project
Other Academics' Involved
Amalka Ranathungage: PhD Candidate, Northumbria University.
Mingyu Zhu: Postgraduate researcher, Northumbria University.
Rana Ayman: PhD Candidate, Northumbria University.
Yomna El-Ghazi: PhD Candidate, Newcastle University.
Related News
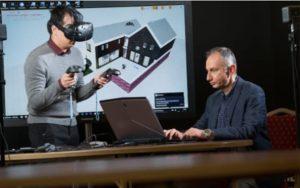
Visualising Carbon Emissions with Virtual Reality
Virtual reality tool that helps people to assess household carbon emissions goes on display at CoP26.
Read Here!
26th October 2021
Useful links
Get in Touch
Get involved with IC3. Contact us or Sign-up to our Newsletter at the bottom of the page.